Introduction
Advanced manufacturing processes like direct energy deposition (DED) are becoming increasingly crucial in aerospace, biomedical engineering, and others. Anyone interested in the future of manufacturing has to grasp the fundamentals of DED. We will examine the principles of directed energy deposition in this extensive guide, covering how it functions, the materials utilized, its applications, and its benefits and drawbacks. By the conclusion of this guide, you will have a thorough grasp of DED and its possible effects on numerous businesses.
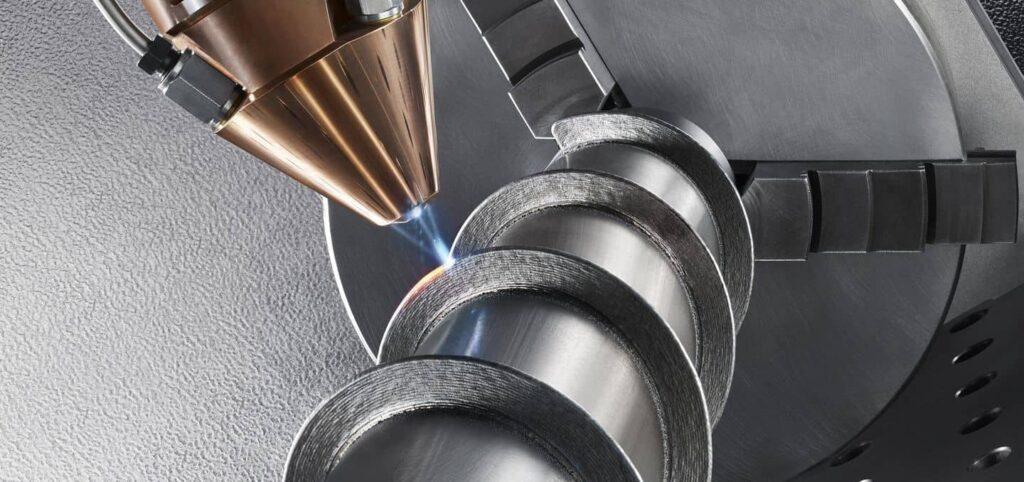
Definition of Direct Energy Deposition (DED)
A form of an additive manufacturing process called directed energy deposition (DED) entails the exact deposition of material utilizing a focused energy source. In Direct Energy Deposition (DED), a nozzle or other deposition device directs a stream of material, and an energy source like a laser or electron beam heats or fuses it. Several industries, including aeronautical and biomedical engineering, use this method to produce intricate 3D structures. Although other additive manufacturing processes like 3D printing are frequently compared to it, Direct Energy Deposition (DED) provides distinct benefits and plays a more significant role in the manufacturing industry.
Importance of understanding the basics of DED
Anyone interested in the future of manufacturing must grasp the fundamentals of Directed Energy Deposition (DED). DED is a very sophisticated and exact manufacturing process that is applied in several fields, including aerospace, biomedical engineering, and others. People may better grasp the benefits and drawbacks of this technology and how it differs from other forms of additive manufacturing by learning the principles of DED. Additionally, as DED spreads throughout the manufacturing industry, experts in industries like engineering and design will increasingly depend on their understanding of this technology. Overall, solid knowledge of DED’s fundamentals may help people stay on the cutting edge of manufacturing technology and contribute to creating new, cutting-edge uses for this technology.
How Direct Energy Deposition Works
Directed Energy Deposition (DED) ‘s operation must be examined to be fully comprehended. DED’s fundamental component is the exact deposition of material with a focused energy source, such as an electron or laser beam. During this process, one must carefully manage the energy supply, material feedstock, and deposition apparatus. This section will focus on the detailed steps involved in DED and the various energy sources and deposition devices employed in this manufacturing process. Readers will have explicit knowledge of how DED operates and the fundamental ideas that guide this sophisticated manufacturing method by the end of this section.
Overview of the DED process
The Directed Energy Deposition (DED) process involves the precise deposition of material layer by layer using a focused energy source, such as a laser or electron beam. The process begins with a digital model of the part or structure to be created. This digital model is then fed into a DED machine, which uses a nozzle or other deposition device to direct a material stream onto a substrate. The material is then melted or fused using the energy source, which is repeated to build up the part layer by layer.
One key advantage of DED is that it allows for the creation of complex three-dimensional structures with high precision. The precise control of the melting and fusion of material in DED is possible because the operator can precisely control the energy source. Moreover, the flexibility of DED is a result of its ability to work with a variety of materials such as metals, polymers, and composites, allowing for the creation of a wide range of parts and structures.
However, the DED process has its challenges. One major challenge is controlling the heat input during the process. The risk of introducing too much heat, which can cause distortion or warping of the part being created, is always present in DED since it involves melting or fusing the material. Moreover, other manufacturing techniques may be faster than the process, which can limit its usefulness in specific applications In general, anyone interested in the future of manufacturing and the potential applications of this advanced technology should understand the DED process.
Types of energy sources used in DED
Diverse energy sources can melt or fuse the deposited material during directed energy deposition (DED). Lasers and electron beams are the two primary energy sources employed in DED. While electron beam-based DED systems employ a high-energy electron beam to perform the same objective, laser-based DED systems use a concentrated laser beam to melt or fuse the material.
Since laser-based DED systems are often more affordable and simpler to use, people employ them more frequently than electron beam-based systems. Lasers are a flexible option for DED since they are accurate and work with various materials. Laser-based devices have a constraint on the penetration depth of some materials, including highly reflecting or transparent ones.
On the other hand, electron beam-based DED systems are beneficial for producing components with high accuracy and precision since they can penetrate a larger spectrum of materials. These technologies, however, are typically more costly and need expert expertise to function well.
Overall, several variables such as the material being used, the level of accuracy sought, and the amount of funding available for the manufacturing process influence the choice of energy source for DED. Anyone interested in the possible uses of DED must thoroughly understand the benefits and drawbacks of each type of energy source.
Benefits and Drawbacks of Laser-Based DED Systems:
Laser-based Directed Energy Deposition (DED) devices are the most widely utilized energy source for DED because of their extreme flexibility and low cost. Laser-based systems’ capacity to work with many materials, such as metals, polymers, and composites, is a significant benefit. Additionally, laser-based technologies offer incredible accuracy and precision for building complex three-dimensional structures. However, there are several restrictions for laser-based devices. For instance, lasers can be less effective in some applications because of their difficulties penetrating higher reflecting or transparent materials. Additionally, the inability of laser-based technologies to create components with high melting temperatures might be a disadvantage in sectors like aerospace or military.
Benefits and Restrictions of DED Systems Based on Electron Beams:
DED systems based on electron beams use high-energy electron beams to melt or fuse the material being deposited. As opposed to laser-based systems, these systems have the significant benefit of penetrating an enormous variety of materials, making them extremely useful for producing components with high accuracy and precision. Additionally, compared to laser-based systems, electron beam-based systems can create components with greater melting temperatures, making them suitable for use in high-temperature industries like aerospace and the military.
However, the cost of electron beam-based systems is often higher than laser-based systems, and their efficient service necessitates specialist knowledge. Furthermore, compared to laser-based systems, electron beam-based techniques may be more complex to maintain and may need more regular calibration. Overall, the selection of an energy source for DED depends on several variables, and everyone interested in the possible applications of DED needs to comprehend the benefits and drawbacks of each type of system.
Comparison Table: Laser-Based vs. Electron Beam-Based Directed Energy Deposition Systems
This table compares the critical differences between electron beam- and laser-based DED systems. Laser-based systems have limits in terms of the materials they can handle and their capacity to make components with high melting temperatures, even though they are often more affordable and straightforward. While more costly and requiring specialized knowledge, electron beam-based systems can penetrate various materials and create components with greater melting temperatures. It is essential to comprehend these variations before selecting the ideal DED system for a particular application.
Characteristics | Laser-Based DED Systems | Electron Beam-Based DED Systems |
---|---|---|
Energy Source | Focused laser beam | High-energy electron beam |
Materials | Metals, polymers, composites, etc. | Metals, ceramics, composites, etc. |
Precision | The high degree of accuracy and precision | The high degree of accuracy and precision |
Versatility | Highly versatile | Limited versatility in terms of material compatibility |
Melting Temperature | Limited ability to produce parts with high melting temperatures | Able to produce parts with higher melting temperatures |
Material Penetration | Difficulty penetrating highly reflective or transparent materials | Can penetrate a wider range of materials |
Cost | Generally more cost-effective | Generally more expensive |
Maintenance | Easier to maintain and operate | More difficult to maintain and may require more frequent calibration |
Comparison to other additive manufacturing techniques
Direct Energy Deposition (DED) is one of the various additive manufacturing processes developed in recent years. Each method has advantages and disadvantages. DED stands out for its capacity to produce massive, complicated pieces from various materials. DED can operate with metals, composites, and polymers. DED is a flexible alternative for several applications. Other popular techniques like FDM, SLA, and SLS have limitations in material compatibility.
However, the application and materials used ultimately determine the best additive manufacturing technology. Therefore, knowing how they vary is essential to make the best option.
Fused Deposition Modeling (FDM) vs. DED Comparison:
Fused deposition modeling (FDM) is another well-liked additive manufacturing method frequently used to produce small-scale items. FDM and DED are two processes that deposit material layers one at a time. DED works with various materials, including metals, polymers, and composites, but FDM primarily uses plastic materials. Fused Deposition Modeling often creates components with lesser accuracy and precision compared to DED. FDM is more suitable for applications that don’t demand high accuracy or detail. Fused Deposition Modeling is a well-liked option for small-scale prototype or hobbyist use. FDM is typically more economical and straightforward than DED.
DED vs. SLA Comparison:
In stereolithography (SLA), a kind of additive manufacturing, a laser or other light source cures a vat of photopolymer resin layer-by-layer. Although both SLA and DED are additive manufacturing methods, there are some significant distinctions between the two. While SLA often utilizes small, detailed parts with high precision, DED is better suited to produce large-scale components with complicated geometries, making it a crucial difference between the two. SLA frequently employs plastic materials, but DED can work with various materials, such as metals and composites. SLA is a superior option for high-detail prototypes or small-batch production runs, although it is often more expensive and time-consuming than DED.
Selective Laser Sintering (SLS) and DED Comparison
SLS (Selective Laser Sintering) is an additive manufacturing process involving layer-by-layer powdered material sintering to produce solid objects. Like DED, SLS can process various substances, including metals, polymers, and composites. But there are some significant distinctions between the two methods. SLS and DED have a crucial difference. SLS often produces components with lower accuracy and precision. Therefore, SLS is more suitable for applications without high accuracy or detail. SLS can be more time- and money-consuming than DED. Hence, SLS is ideal for small-scale production runs or prototyping than large-scale manufacturing.
In general, the choice of additive manufacturing process relies on the particular application and the materials used. Picking the best approach requires a grasp of the distinctions between the different techniques.
Materials Used in Direct Energy Deposition
Direct energy deposition (DED), a highly adaptable additive manufacturing technology, can use a wide variety of materials. DED can work with metals, polymers, and composites, unlike traditional manufacturing processes. Engineers and manufacturers commonly use DED to produce a range of products, from aircraft components to medical equipment. DED allows the use of various materials, making it an excellent choice. This section will focus on the most common DED materials and their unique characteristics that make them suitable for this advanced manufacturing technique.
Types of materials used in DED
The capacity of Direct Energy Deposition (DED) to function with various materials gives it flexibility. DED frequently utilizes metals, polymers, and composites. Manufacturers prefer metals, including titanium, aluminum, and stainless steel, due to their strength, durability, and corrosion resistance. Because they are lightweight and flexible, polymers like ABS, nylon, and polypropylene are perfect for producing parts that need to combine strength with flexibility. The automotive and aerospace industries widely use composite materials like carbon fiber and fiberglass because of their excellent strength-to-weight ratios. In addition to these often-used materials, DED can also deal with ceramics. The ability to deal with such a wide range of materials is one of the primary advantages of DED, making it a versatile alternative for many applications.
Metals
Metal is one of the most popular materials in Direct Energy Deposition because of its power, toughness, and corrosion resistance. Titanium, aluminum, and stainless steel are just a few of the many metals that DED can deal with. These metals’ propensity for withstanding severe stress and a wide range of temperatures makes them widely employed in aerospace, automotive, and medical applications. DED may also employ nickel-titanium alloys, which have unique shape memory qualities that make them valuable in medical devices like stents and orthodontic wires. One of the key benefits of using metals in DED is the ability to precisely manufacture complicated geometries, making it a preferred option for fabricating sophisticated parts like turbine blades and engine components.
Advantages
- High strength-to-weight ratio
- High durability and corrosion resistance
- Ability to withstand high stress and extreme temperatures
- Ability to create complex geometries with high precision
Disadvantages
- High cost for certain metals
- Limited to certain types of metals that can be used in DED
polymers
They are perfect for producing pieces that need a balance of strength and flexibility since they are lightweight and flexible. DED can work with various polymers, including ABS, nylon, and polypropylene. Manufacturers frequently use these polymers in consumer products like toys and electronics due to their low cost and ease of use. In addition, DED can employ more sophisticated polymers such as PEEK and ULTEM, which have excellent strength-to-weight ratios and are often used in aerospace and medical applications. One of the key advantages of employing polymers in DED is the ability to make components with complicated interior geometries, making it a preferred choice for constructing medical implants and other specialized devices. Polymers are a flexible choice for producing pieces with distinctive visual features since they are simple to tailor with various colors and textures.
Advantages
- Low cost and ease of use
- Lightweight and flexible
- Ability to create complex internal geometries
- Customizable with different colors and textures
Disadvantages
- Limited strength compared to metals and composites
- Can deform under high stress or temperature
composites
Composites, which have excellent strength-to-weight ratios, are a common material used in Direct Energy Deposition (DED). Manufacturers create composites by mixing two or more separate substances to produce a substance with unique qualities. Two popular types of composites utilized in DED are carbon fiber and fiberglass. Aerospace and automotive applications often use carbon fiber composites for their excellent strength and rigidity. Fiberglass composites, on the other hand, are well-liked for constructing items that require high-impact resistance due to their robust and long-lasting nature. In addition to these frequently used composites, DED can also deal with less common composites like graphene and carbon nanotube composites, which have extraordinary strength and conductivity. Engineers and manufacturers may build items with distinctive qualities that are impossible to attain with conventional materials thanks to the ability to work with composites in DED.
Advantages
- Superior strength-to-weight ratio
- Ability to create parts with unique properties
- High impact resistance
- Improved strength and fracture resistance with composite materials
Disadvantages
- High cost for certain composites
- Limited to certain types of composites that can be used in DED
ceramics
Due to their strength, hardness, heat resistance, and wear resistance, ceramics provide an excellent direct Energy Deposition (DED) material. Ceramics like alumina, zirconia, and silicon carbide, which offer increased strength and fracture resistance, are widely employed in high-temperature and high-stress applications, including aerospace and medical equipment. DED can also use ceramic composites. One of the main advantages of using ceramics in DED is their ability to withstand extreme environments that other materials cannot. But since they are fragile and prone to breaking, ceramics are more challenging than other materials. Despite these difficulties, ceramics are crucial for particular DED applications due to their distinctive features.
Advantages
- Hardness, strength, and resistance to heat and wear
- Ability to withstand extreme environments
- Improved strength and fracture resistance with ceramic composites
disadvantages
- Brittle nature and susceptibility to cracking
- Difficult to work with compared to other materials.
Applications of Direct Energy Deposition
Direct Energy Deposition (DED) is a flexible additive manufacturing method that has become more well-known in various sectors because of its capacity to create intricate and high-quality parts. DED has established itself as a dependable and effective manufacturing process for creating components with distinctive geometries and qualities, from aerospace to biomedical applications. In this part, we’ll look at the numerous ways DED is used in various fields and the advantages each one offers. Manufacturers and engineers may more effectively use DED to produce high-performance components at a reasonable cost by being aware of its variety of applications and advantages.
Overview of industries that use DED
Direct Energy Deposition (DED) has found various applications in various industries due to its ability to produce high-quality, complex parts. Here is an overview of some of the industries that utilize DED technology:
Aerospace and Defense
The aerospace and defense industry is one of the most significant users of DED technology. Companies such as GE Aviation use DED technology to produce turbine blades for aircraft engines. They choose this technology because it can create parts with intricate geometries and using multiple materials, and the properties of the parts can be tailored to meet specific requirements. DED is a technology that is utilized to produce parts such as turbine blades, engine components, and aircraft structures. The DED process allows for the production of blades with intricate internal cooling channels that improve the performance and efficiency of the engine.
Biomedical
DED technology is also used in biomedical applications to produce complex implants and medical devices. DED can produce high-quality parts, allowing for a customized fit for patients. Additionally, the ability to use biocompatible materials makes DED a superior technology for creating implantable devices. DED technology has been used in orthopedic surgery to produce customized patient implants. An example of this is the use of DED to produce hip replacement implants that fit each patient’s unique anatomy.
Automotive
The automotive industry also uses DED technology to produce high-performance parts such as engine components, exhaust systems, and suspension. DED can create parts with a high strength-to-weight ratio, which is essential for improving fuel efficiency and performance. Ford Motor Company has utilized DED technology to produce components such as cylinder heads and engine blocks. DED technology allows for making parts with complex geometries and optimized material properties, improving fuel efficiency and performance.
Tooling and Manufacturing
DED technology is also utilized in tooling and manufacturing industries to produce molds, dies, and other tooling components. The use of DED in tooling and manufacturing can reduce lead times, increase productivity, and lower costs. Optomec has utilized DED technology to produce molds and dies for the injection molding industry. DED technology in tooling and manufacturing can reduce lead times, increase productivity, and lower costs for manufacturers.
These are just a few examples of industries that use DED technology. As technology evolves and improves, it is expected to find even more applications in various industries.
Advantages and Challenges of Direct Energy Deposition
Direct Energy Deposition (DED) technology offers several advantages over traditional manufacturing processes. Here are some of the advantages of DED:
- Design Flexibility: DED allows for producing complex geometries that are difficult or impossible to produce using traditional manufacturing processes. This opens up new design possibilities and enables the production of parts with improved performance.
- Material Versatility: DED technology can be used with various materials, including metals, polymers, ceramics, and composites. This versatility allows for the production of parts with tailored properties to meet specific requirements.
- High Deposition Rates: DED can deposit material at rates much higher than traditional manufacturing processes, resulting in shorter production times and increased productivity.
Despite these advantages, there are also several challenges associated with DED technology. Some of the challenges include the following:
- Process Control: DED is a complex process that requires precise control of several parameters, including temperature, powder flow rate, and laser power. Any deviation from the optimal settings can result in defects in the final part.
- Post-Processing: Parts produced using DED often require post-processing, such as machining or polishing, to achieve the desired surface finish and dimensional accuracy. This post-processing can add time and cost to the production process.
- Equipment Cost: DED equipment can be expensive, especially for high-end systems with advanced features and capabilities. This high cost can be a barrier to entry for smaller manufacturers or companies with limited budgets.
Overall, while DED technology offers several advantages over traditional manufacturing processes, it is essential to consider the associated challenges and limitations when deciding whether to adopt this technology.
Advantages of DED compared to other manufacturing techniques
Technology | Main Advantages | Main Disadvantages |
---|---|---|
Direct Energy Deposition (DED) | Design flexibility, material versatility, high deposition rates | Process control, post-processing, equipment cost |
Fused Deposition Modeling (FDM) | Low cost, ease of use, good for low-volume production | Limited material selection, poor surface finish, low dimensional accuracy |
Stereolithography (SLA) | High accuracy, good surface finish, a wide range of materials | Limited build volume, high equipment cost, and post-processing required |
Selective Laser Sintering (SLS) | Wide range of materials, good strength, and durability, good surface finish | High equipment cost, post-processing required, limited design flexibility |
Binder Jetting (BJ) | Low cost, good for large parts, high printing speed | Poor mechanical properties, limited material selection, and post-processing required |
It’s important to note that this chart only compares different technologies and that each technology’s performance may vary based on the particular application and use situation. In the end, the technology used will rely on the specific demands and specifications of the current project.
Conclusion
In conclusion, Direct Energy Deposition (DED) is a versatile and promising additive manufacturing technology offering many advantages over traditional manufacturing methods. DED enables a high degree of design flexibility, material versatility, and part complexity by using a focused energy source to melt and deposit layer by layer. However, as with any technology, there are challenges and limitations associated with using DED. In this section, we will summarize the key points discussed in this article and provide some final thoughts on the future of DED and its potential impact on manufacturing.
Summary of key points
- Direct Energy Deposition (DED) is a type of additive manufacturing that uses a focused energy source to melt and deposit material layer by layer.
- DED can use various energy sources, including lasers, electron beams, and plasma arcs.
- The DED process uses a deposition nozzle or other tool to place the melted material onto the workpiece precisely.
- DED can use various materials, including metals, polymers, composites, and ceramics.
- DED offers advantages over traditional manufacturing methods, including greater design flexibility, material versatility, and part complexity.
- DED is used in various industries, including aerospace, automotive, and medical.
- DED’s key challenges and limitations include material selection and compatibility, process control, and post-processing requirements.
- DED is still a relatively new technology with ongoing research and development, and its full potential is yet to be realized.
Overall, DED is a promising technology that offers many benefits for manufacturing, but it also requires careful consideration and expertise to achieve optimal results. We can expect to see even more applications and innovations as technology advances.
Future of DED in manufacturing and other industries
Direct Energy Deposition (DED) has a promising future ahead of it in the industrial sector and other sectors. As technology develops and improves, DED will become even more widely used in several industries, including aerospace, automotive, and healthcare.
Creating better and more advanced materials compatible with technology is one of the main areas of emphasis for the future of DED. This involves developing novel metal alloys, polymers, and composites that can be employed with DED and investigating brand-new uses for current materials.
Process control and automation will be another area of emphasis for DED in the future. The demand for automated procedures and quality control systems that can guarantee repeatable and dependable outcomes is increasing as DED is more frequently used. This entails the creation of sophisticated feedback and monitoring systems capable of real-time mistake detection and correction.
DED has potential uses outside of manufacturing, as in the building and architectural sectors. DED can completely change how we design and construct buildings and other structures by making it possible to create intricate and personalized systems and components.
Overall, DED’s future is promising, and many intriguing possibilities are ahead. In the years to come, we anticipate seeing even more uses and breakthroughs as technology develops and improves, which will result in a manufacturing sector that is more productive, economical, and sustainable.
FAQ
DED is an additive manufacturing technology that uses energy from a focused heat source to melt and fuse materials together to create a 3D object.
DED offers several advantages, including the ability to produce large and complex parts, the use of a wide range of materials, and the ability to repair and modify existing components.
Some of the challenges and limitations of DED include the high cost of equipment, the need for skilled operators, the potential for porosity and other defects, and the limited range of materials that can be used in the process.